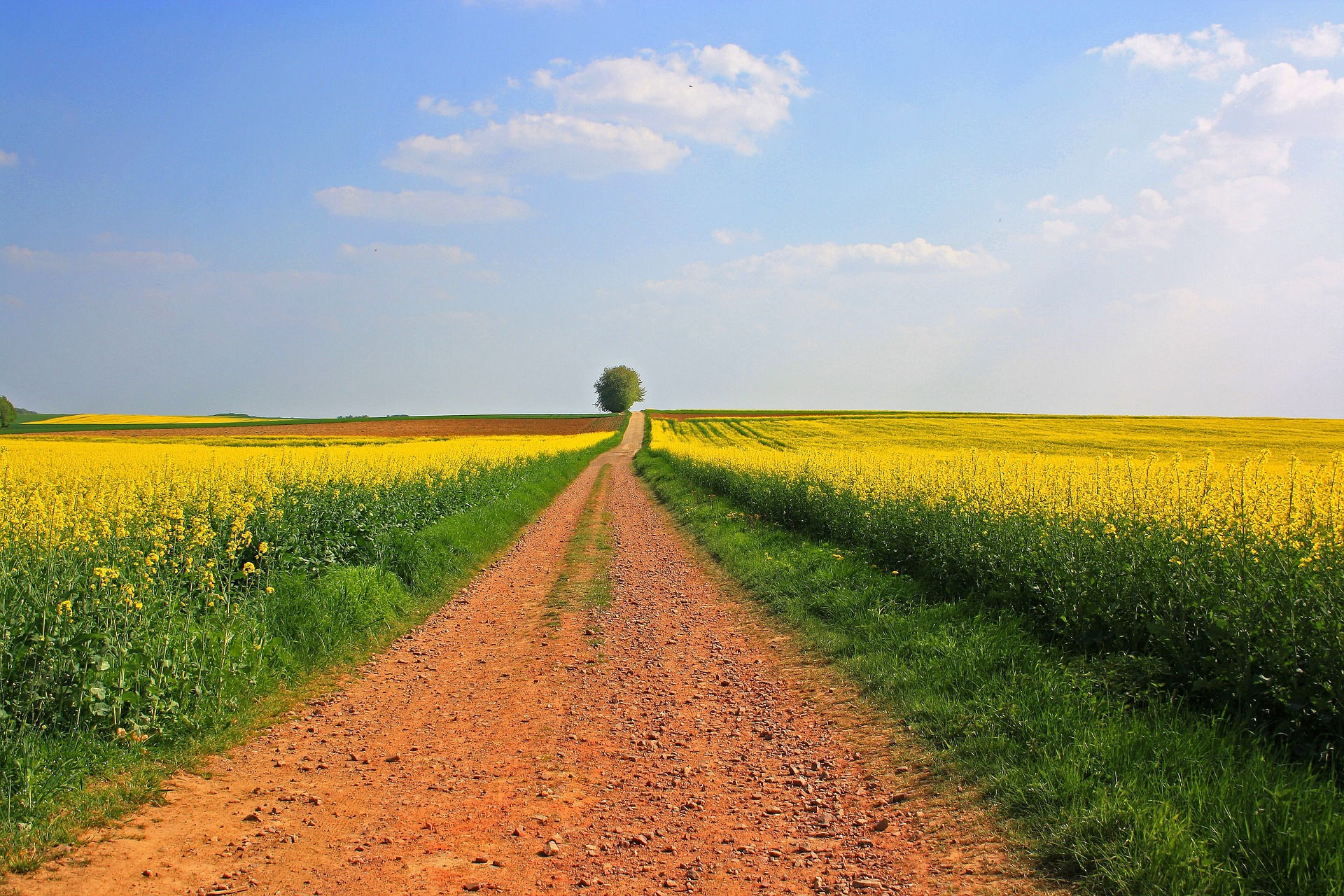
What's New
Mouth Sensor Can Measure the Salt in Every Potato Chip You Eat
People with high blood pressure could get real-time data about sodium intake
By Eliza Strickland
If you’re one of the approximately 36 million adults in the United States with high blood pressure (also called hypertension), your doctor may have lectured you about the importance of reducing your sodium intake. Maybe you listened, and maybe you even intended to follow your doctor’s advice.
But sodium, the primary component of table salt, is everywhere in our modern diets—in snack foods, in restaurant meals, even in beverages—and your good intentions probably didn’t get you very far.
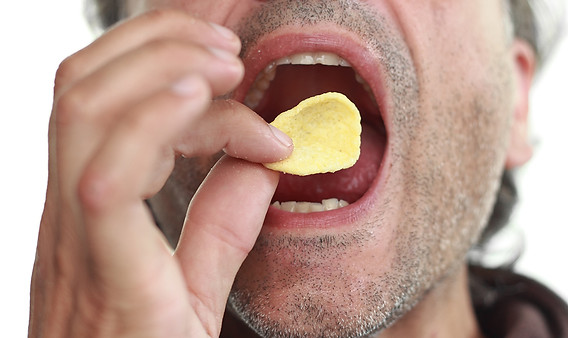
To tackle this problem, researchers at the Georgia Institute of Technology have invented a flexible electronic sensor that can be embedded in a dental retainer for real-time monitoring of sodium intake. The device, which they described in the journal PNAS, could send info to your phone, giving you instant data about whether you’re busting your low-sodium diet.
Whether you decide to change your diet based on that data is another question entirely. But getting the data is the first step.
W. Hong Yeo, an assistant professor of micro and nano engineering who led the research team, says it would also be possible to stick the sensor directly to the tongue or the roof of the mouth, or to laminate it onto a tooth. The soft retainer they used in this experiment was just phase one. “For the first prototype device, we wanted to offer easy handling and cleaning capability via the integration with a soft retainer,” he said.
Yeo says the biggest challenge was making the entire electronic device soft, flexible, and comfortable enough to wear in the mouth. So the team designed a chip that uses stretchable circuits mounted on an ultrathin porous membrane.
Its power source is a rechargeable micro-coin battery (measuring 6.8 mm in diameter), which could continuously monitor real-time sodium intake for 12 hours. Yeo thinks that’s a long enough battery life for practical use, as a wearer could put in the retainer just at meal times and recharge it at night. But his team is considering removing the battery in the design of an even smaller sensor, which could receive power from an external source via inductive coupling.
The sodium sensor itself uses inexpensive materials that respond to the presence of sodium ions. To test the gadget, Yeo’s team first had people take sips of water with various concentrations of salt. When it proved adept at measuring those sodium levels, they moved to a harder challenge: real food and drink. The test subjects took a gulp of veggie juice, slurped up a mouthful of chicken noodle soup, and crunched down on a potato chip.
Nanobots Glide Through Living Cells
Rotating magnetic fields precisely steer the nanomachines, tracing letters in cytoplasm
By Megan Scudellari
Posted 13 Apr 2018 | 17:00 GMT
It’s time to let go of the idea that nanomachines are simply life-size technology shrunk down to a very small size, vis-à-vis the 1960s movie Fantastic Voyage.
In fact, a lot of nanotechnology is much, much cooler. That includes a corkscrew-shaped nanomotor described this week in the journal Advanced Materials. Using small, rotating magnetic fields, researchers steered the itty bitty machines inside of living cells to trace the letters “N” and “M,” corresponding to the word nanomotor.
“We not only showed their motion inside a cell, we have engineered a strategy to move them in controlled fashion” and without hurting the cells, said the paper’s coauthor Malay Pal, of the Indian Institute of Science in Bangalore, in an email to IEEE Spectrum.
Other popular forms of nanomotors include nanorods propelled by acoustic or electrical means. These tiny spinning sticks can churn up the inside of a cell, but it is hard to control their direction. Ultrasound-propelled nanorods are also limited because when ultrasound is applied, cells begin to float. That makes it impossible to experiment on cells stuck to a surface, which is the normal state of most cells, notes Pal. Ultrasound may also induce stress in living tissue, causing unintentional damage.
Taking another tack, Pal’s Ph.D. advisor, Ambarish Ghosh, a researcher at the Center for Nano Science and Engineering, began to experiment with helical nanostructures controlled by magnetic fields, which do not lift or stress cells. Ghosh, Pal, and their collaborators fabricated the nanomotors out of silica, then coated them with iron. The team evaluated two sizes of these nanomotors (with diameters of 400 nanometers and 250 nanometers) in three types of living cells. Most cells took up a single nanomotor, while some incorporated several.
The researchers placed a dish with the cells within a magnetic coil under a microscope. Then, by rotating the magnetic field, they were able to control and track the movement of the nanomotors inside the cells. The smaller, 250-nanometer motors were easier to steer than the larger ones, notes Pal.
The work is at an early stage, but “these tiny machines have tremendous potential in applications like targeted drug delivery, nano sensing, therapeutic[s and] nano surgery,” said Pal. In January, the team showed they could use the helical nanomachines as sensors to measure the viscosity of a fluid, and as nanotweezers to pick up, transport, and release objects on the nanoscale.
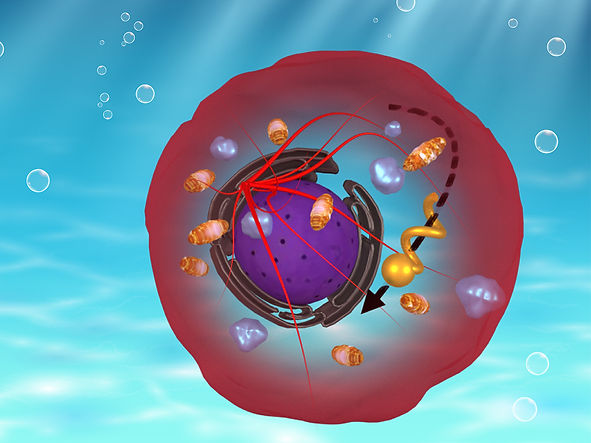